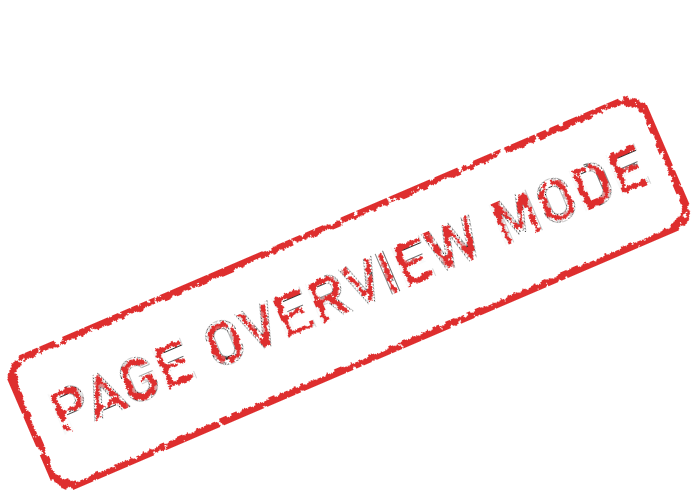
Electrical power
The ancient Greeks were said to have discovered static electricity by rubbing fur on other materials.
Scientists were predicting the effects of electricity in the early 1600s. Benjamin Franklin’s kite flying experiment of 1752. showed the relationship between lightning and electricity. In 1786., Luigi Galvani, an Italian medical professor, discovered that a metal knife touching the leg of a dead frog caused violent twitching. He proposed that the frog’s leg must contain electricity. In 1792., Alessandro Volta disagreed. He proposed that the discovery was centered around dissimilar metal of the knife. When moisture came between them, electricity was created. This discovery led Volta to invent the first modern electric battery, a galvanic cell. The new discovery was revolutionary, until then all electricity discoveries had centered around static electricity and discharged sparks. Volta showed that this new kind of electricity, which flowed like water, could be made to travel from one place to another in a controllable way. In 1831., Michael Faraday, from London, discovered the next major breakthorough. He found that when a magnet was moved inside of a coil of wire, electricity was produced. Where Volta had created an electricity source via a chemical reaction, Faraday created one through mechanical motion. He made a relatively simple experiment in nature - he made a coil by wrapping wire around a paper cylinder, connected the coil to a galvanometer and observed it when moving a magnet back and forth between the cylinder, observing magnet stationary and magnet in movement. When the magnet was stationary, no current was created in the wire and thus no voltage was observed at the ends of the wire; when the magnet was moving Faraday observed an induced current through the wire.
Faraday’s experiment was termed electromagnetic induction, since a magnet was inducing the electricity on the wire.
Soon, Thomas Edison, began thinking about uses for electricity. His creation of a small incandescent lamp in 1879. which was suitable for indoor use led to his creation of a generation station in lower Manhattan, in New York City. By the mid 1880s, cities all over America yearned for their own electrical generation stations so they too could use Edison’s incandescent light to illuminate the insides of their buildings.
Using Faraday’s principles of electromagnetic induction, Edison created a generator capable of producing DC, or direct current. One of Edison’s employees, Nikola Tesla, had been working on a generation machine of his own that produced what Tesla called AC, or alternating current.
George Westinghouse, saw the potential for electricity and created his own company. He purchased the rights to Tesla’s invention and soon took on Edison in an epic battle to decide which machine was better capable of producing electric power.
AC power generation
Whether we’re dealing with AC or DC, electrical power generation as the result of some mechanical motion is generally handled by two principal components:
· field (that is used to create current)
· armature (that carries the current that is being generated)
To create AC power, we can start with the idea proposed by Faraday - a moving magnet and coil of wire produce electric potential. Similarly, a moving coil of wire in a magnetic field also produces electric potential. If we were constantly to rotate this coil of wire, the induced voltage would change in sinusoidal fashion. The voltage constantly cycles between some peak values, when the coil is perpendicular to the magnet. Along the way, when the coil is parallel to the magnet, the induced voltage is 0. It’s also very important to note that the coil must be rotating for this voltage to be induced. If at any time the rotation stops, even if the coil stays oriented perpendicular to the magnet, the induced voltage will drop to zero.
Now, we need a way to get this induced voltage out of the ends of the coil of wire into something useful. The dilemma is that if the coil of wire is constantly rotating, it becomes difficult to connect the ends of the wire to anything practical since it would also have to rotate with the coil.
The easiest fix for this is to use a device called a slip ring which is basically an electrical connector that can rotate. Internally, the slip ring is actually a graphite brush that is in constant contact with a metal disk. As the disk turns, the brush is always in contact with it. This allows the current to constantly flow between the brush and the disk no matter if the disk is turning or not.
One downside to using slip rings is that their constant motion means there is some friction between the brushes and the metal rings. Over time, the brushes wear out and must be repaired or replaced. This means that there is some maintenance required for slip ring based devices.
So, connecting the slip rings to the ends of the coil of wire allows the coil to continually rotate while allowing the wires coming out of the generator to remain stationary.
DC power generation
DC power generation is similar to that of AC power generation. A coil of wire is rotated within the presence of a magnetic field. This in turn induces current in the wire. How that current is used, however, is different than that with AC. Instead of using slip rings, like with AC, a DC generator has its wires attached to a commutator. The commutator is a type of rotating switch that allows the current flow to reverse direction in the wires. What happens in the DC generator is the same as the AC generator for the first part of the cycle. In the second part of the cycle, things change; with the commutator action as the rotor continues to spin through this section, current again flows in the direction it was flowing before. The end result is that a DC generator flips the flow of current when it would normally be negative in an AC generator. This means that the current flow is always in the same direction.
Other than 2-pole generator, the 4-pole generator, however, has a much more stable output. A 6-pole or 8-pole motor would have an even better output. Additional poles, though, require a more complex and thus more expensive commutator.
Motors and generators
Motors and generators are basically the same thing, except that power flow goes from electrical to mechanical in a motor and mechanical to electrical in a generator. Generators take mechanical motion and turn it into electricity. However, the opposite transformation, going from electricity into mechanical motion, is also commonly desired and that is what a motor does. The same principles we’ve described for generators apply to motors as well, particularly for DC motors. However, many AC motors today, instead, use a fixed armature with a rotating set of magnets (electromagnets or permanent magnets). Current flowing into the fixed armature (the stator) creates a magnetic field that induces current in conductors in the inner rotating part (the rotor). The induced current in the rotor creates another magnetic field that counteracts to the original magnetic field in the stator. The two magnetic fields oppose each other, causing the rotor to turn.
DC generators use commutators to get the DC power out of the generator. These commutators utilize brushes that transfer the power from the rotating part of the generator to the stationary part. When the generated voltage becomes very high (near 1000V) it can cause a spark between a brush finger and the shaft when contact is lost. This sparking isn’t good for the brushes or the DC motor, which means that it becomes expensive to utilize a DC motor at high voltages.
DC power generation worked well to power incandescent lights, which were about the only things needing electrical power at the Edison's time, but it quickly became apparent that the downside to Edison’s DC power was in the distribution. A low DC voltage was impractical to transmit across long distances because long power lines did not have neglibile resistance. High DC voltages were difficult to generate because of sparking that would occur in the armature of the generator. DC power was also very difficult to change to higher or lower voltages.
The solution proposed by Edison was to have generation facilities near by the places where it would be utilized. Each needed voltage would be transferred on a separate wire.
Westinghouse’s AC power handled higher voltages much more readily. The higher AC voltage was easier to transmit over longer distances because the amount of electrical power loss in the power lines was minimal compared to the transmitted voltages. The main advantage, though, of this form of distribution was the easy ability to transform AC power from higher voltages to lower ones, using transformer. AC power now could be generated at a centralized station and distributed over long distances, being transformed down to lower voltage AC power at its destination.
Talking about power lines, which are thick cables strung over very long distances, the resistance of the wire becomes a factor, it is not negligible. This means that as we’re using the electrical power lines to send current from a generating station to customers, some of the power we generate is lost due to the resistance of the power lines (power lost as heat in the transmission lines, which we want, of course, to minimize as much as possible). So, for a fixed power requirement (from consumption needs that we are trying to address), if we were to increase our generated voltage it would reduce the amount of current that would have to flow into the wire (power = voltage x current). Ohm’s Law states that less current flowing through the resistive wire means a smaller voltage drop across the wire, which means less power is lost in the wire. This means more power can be delivered to the final destination.
The power generation station at Niagara Falls, by Westinghouse and Tesla worked wonderfully with the power being distributed to Buffalo, New York at a distance of over 20 miles. Niagara Falls is still one of the largest electrical power generation stations in the United States. AC became the practical standard for power generation and distribution today.
When we mention AC, for example, we’re referring to the alternating current that passes through a fixed resistance. This then implies that there must be a related alternating voltage that creates the alternating current. Similarly with DC, for a fixed resistance there is a steady voltage that must be supplying the current. It’s very common to refer to voltages as DC or AC.
Both AC and DC are methods for distributing power. As the power requirements of the load changes, both attempt to maintain their voltage values. For example, in our house, the AC voltage coming in is always at a fixed 230V AC, relatively. Over time, the power requirements of our house change as electrical appliances turn on or off. This means that the resulting alternating current generated by this voltage may be higher or lower at any given point in time. If no electricity is being used, then no current flows into our house. But the 230V AC is still present.
While a DC voltage doesn’t change, AC voltage is always alternating back and forth in a fixed way. The voltage is constantly changing from some positive value, down to 0, then down further into a negative value. It then comes back up and repeats the cycle over and over again. Since the graph of voltage over time looks somewhat like a wave of water, it’s called a waveform. The height of the voltage, from midpoint to peak, is called the amplitude, the time between two waves is known as the period. The frequency is waveform cycles per second, the period is reciprocal of frequency. The wavelength is the physical distance between waves; the period is the time between successive peaks in the wave, the wavelength is the distance separating two such peaks. In order to figure this out, we have to know how fast the wave is moving, wavelength = velocity/frequency.