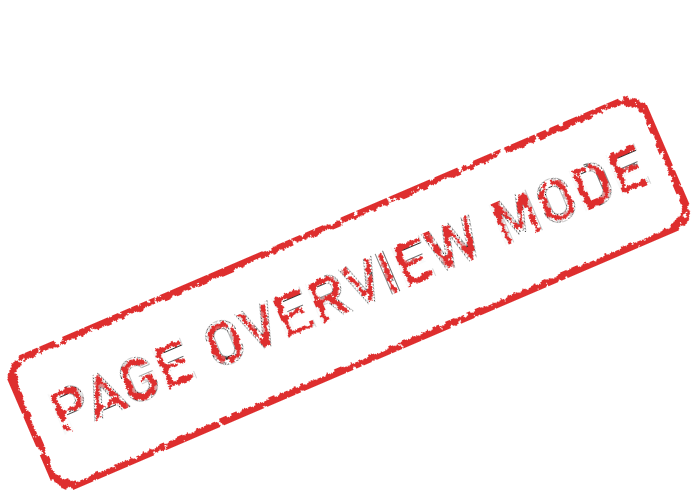
Magnetic fields and circuits
Magnetic materials
All materials can be classified as being magnetic or non-magnetic, depending upon the degree to which they exhibit magnetic effects.
The vast majority of materials fall into the latter group, which may be further classified into diamagnetic and paramagnetic materials. The magnetic properties of these materials are very slight, and extremely difficult even to detect. Thus, for practical purposes, we can say that they are totally non-magnetic. The magnetic materials (based on iron, cobalt and ferrites) are the ferromagnetic materials, all of which exhibit very strong magnetic effects.
Magnetic fields
Magnetic fields are produced by permanent magnets and by electric current flowing through a conductor. Same as the electric field, a magnetic field may be considered as being the medium by which forces are transmitted. A magnetic field is also represented by lines of force or magnetic flux, and these lines have certain characteristics:
(1) They always form complete closed loops.
Unlike lines of electric flux, which radiate from and terminate at the charged surfaces, lines of magnetic flux also exist all the way through the magnet.
(2) They behave as if they are elastic. That is, when distorted they try to return to their natural shape and spacing.
(3) In the space surrounding a magnet, the lines of force radiate from the north (N) pole to the south (S) pole.
(4) They never intersect.
(5) Like poles repel and unlike poles attract each other.
Permanent magnets have the advantage that no electrical supply is required to produce the magnetic field, but because of some disadvantages in practice - they are relatively bulky, the strength of the field cannot be varied, over a period of time they tend to lose some of their magnetism; more convenient method is required to produce a magnetic field.
Electric current, except from heating effect, also produces a magnetic field. The strength of this field is directly proportional to the value of the current. Thus a magnetic field produced in this way may be turned on and off, reversed, and varied in strength very simply. A magnetic field is a vector quantity.
The field pattern produced by a current flowing through a straight conductor is illustrated in the figures below.
The current flowing away from the observer is marked by a cross, and current towards the observer is marked by a dot. The direction of the arrows on the flux lines can be determined by considering the X as the head of a cross-head screw. In order to drive the screw away from us, the screw would be rotated clockwise. On the other hand, if we were to observe the point of the screw coming out towards us, it would be rotating counterclockwise. This convention is called the screw rule, and assumes a normal right-hand thread.
Magnetic flux actually extends the whole length of the conductor, in the same way that the insulation on a cable covers the whole length. In addition, the flux pattern extends outwards in concentric circles to infinity. However, as with electric and gravitational fields, the force associated with the field follows an inverse square law. It therefore diminishes very rapidly with distance.
The flux pattern produced by a straight conductor can be adapted to provide a field pattern like a bar magnet. This is achieved by winding the conductor in the form of a coil, and the arrangement is known as a solenoid.
The magnetic circuit
A magnetic circuit is all of the space occupied by the magnetic flux. On the next figure we have an iron-cored solenoid, supplied with direct current, where the resulting flux pattern is shown.
The spacing of the lines within the iron core is uniform, whereas it varies in the air space. Thus there is a uniform magnetic field in the core and a non-uniform field in the rest of the magnetic circuit.
For a simplicity of design and analysis of magnetic circuit, it is more convenient if a uniform field can be produced. This may be achieved by the use of a completely enclosed magnetic circuit.
One form of such a circuit is an iron toroid, that has a current carrying coil wound round it. A toroid is a ‘doughnut’ shape having either a circular or a rectangular cross-section and provided that it has a uniform cross-section then the field contained within it will be uniform.
The magnetic flux is what causes the observable magnetic effects such as attraction, repulsion etc. The unit of magnetic flux is the weber (Wb), named after German physicist, Wilhelm Eduard Weber (1804–1891).
The number of webers of flux per square metre of cross-section of the field is defined as the magnetic flux density (B), which is measured in tesla (T), named in honour of the Serbian-American engineer, Nikola Tesla (1856–1943):
Magnetomotive force (mmf)
Magnetic flux is, similarly to current in electric circuit which is due to the existence of an emf, due to the existence of an mmf. It is much more easier and simple, other then concept of an mmf for permanent magnets, to consider the flux being produced by current flowing through a coil, which is the case for most practical magnetic circuits.
Each turn of the coil makes a contribution to the total flux produced, so the flux must be directly proportional to the number of turns on the coil. The flux is also directly proportional to the value of current passed through the coil.
So, the mmf is the product of the current and the number of turns, F=N‧I [ampere turn].
Magnetic field strength
Magnetic field strength is magnetic equivalent to electric field strength in electrostatics. We showed earlier that electric field strength is the same as potential gradient, and is measured in volt/metre. Since mmf and emf are comparable quantities, mmf can be considered as the magnetic circuit equivalent of electric potential. Hence magnetic field strength is defined as the mmf per metre length of the magnetic circuit.
• l - the mean or average length of the magnetic circuit
Example:
A current of 400 mA is passed through a 550 turn coil wound on a toroid of mean diameter 8 cm. Calculate the magnetic field strength.
Solution: