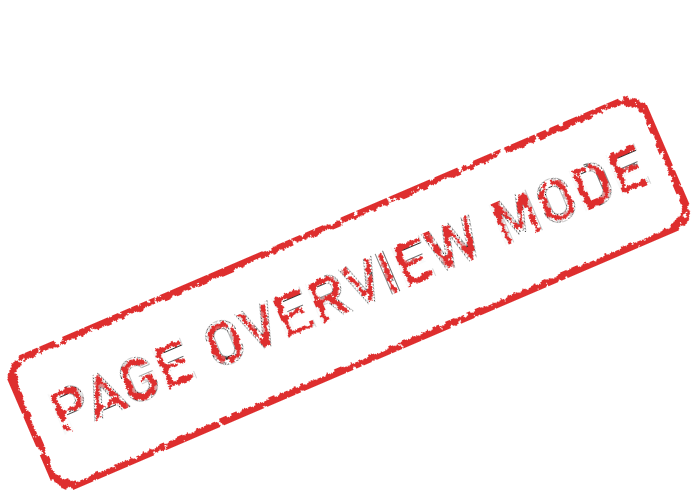
In 1911., the New Zealander Sir Ernest Rutherford suggested that an atom consisted mainly of ‘empty space’, with a heavy ‘nucleus’, around which electrons moved in different orbits – similar to the way in which the planets circle the Sun.
However, this ‘planetary’ model didn’t quite account for the way in which the electrons appeared to behave. The planets in our solar system are continuously losing the energy they need to maintain themselves in their orbits. This causes those orbits to get a little smaller over time, but this didn’t seem to be the case with electrons; their orbits weren’t getting any smaller, which suggested that electrons simply couldn’t be ‘gradually’ losing energy! Scientists were intrigued about what prevented an electron from gradually losing its energy and eventually spiralling into its nucleus. The eventual answer to this riddle resulted in a completely new way at looking at physics: something called ‘Quantum Mechanics’, the idea of the German physicist, Max Planck (1858–1947).
Quantum Mechanics explains that an electron’s energy can only change in discrete (distinct) amounts, which are called ‘quanta’. The radius of an electron’s orbit (called a ‘shell’) depends on the energy level of that electron; the higher its energy level, the greater the radius of its orbit. If its level of energy cannot change gradually, then neither can the radius of its orbit. So, if the energy levels of electrons can only exist in discrete amounts (‘quanta’), then their orbits can only exist at discrete distances from an atom’s nucleus. Quantum Mechanics explains that electrons cannot exist between these orbits.
To move from an inner orbit or shell, to an outer one, an electron must gain a quantum (discrete amount) of energy. Similarly, to move from an outer shell to an inner shell, an electron must lose that quantum of energy.
In 1913. the Danish physicist Niels Bohr combined Rutherford’s model of the atom with Planck’s theory of ‘quanta’ and proposed a new model of the atom.
Bohr's model of the atom
Bohr’s model of the atom consists of a nucleus, surrounded by up to seven orbits called electron shells (labelled k, l, m, n, o, p and q). The electron capacity of each shell is determined by the expression, 2n2 – where n represents the sequence of the shell, counting outwards from the nucleus. So, the innermost (‘k’) shell has a capacity of 2 (2x12), the next shell has a capacity of 8 (2x22), the next a capacity of 18 (2x32), and so on.
Bohr’s model of the atom had its limitations, which prevented it from explaining later discovered behaviours, and other physicists have continued to develop that model further. In 1932, for example, James Chadwick proposed that the atom’s nucleus was not a single heavy mass but, instead, made up of two types of ‘elementary particle’: positively charged protons, and neutrons which carry no charge at all. And it was the number of protons within the nucleus that determined all of nature’s elements.
But there remained many questions that still needed answering. And, today, physicists working for organizations such as CERN (the European Organization for Nuclear Research) are continuing to unravel the true nature of the atom, and have either discovered, or believe in the existence of new sub-atomic particles...
Today’s scientists no longer think of the atom in terms of Bohr’s model – the present model is still evolving, but Bohr’s model of the atom is perfectly adequate to help us understand the nature and behaviour of electricity and how some atoms provide the charge carriers necessary for the phenomenon called an electric current.
Matter is anything, such as a solid, liquid or gas, that has weight (mass) and occupies space. For anything to occupy space, it must have volume. And matter is made up of tiny particles, called atoms. Matter that consists entirely of identical atoms is termed an element, and there are 92 naturally occurring elements, together with others that are manmade. Examples of elements include hydrogen, helium, oxygen, carbon, copper, uranium, etc. Matter that consists of a combination of different atoms is termed a compound, and these combinations of atoms we call molecules. Water, for example, is a compound made up of molecules, each of which comprises two atoms of hydrogen (symbol: H), together with one atom of oxygen (symbol: O) – hence its chemical symbol: ‘H2O’. The structure of a molecule determines whether an element or a compound is a solid, a liquid or a gas. With solids, molecules form rigid, crystal-like, structures. With liquids, the molecules are not bound together as rigidly, resulting in the fluid nature of a liquid. And with gases, the molecules drift apart from each other, and disperse to fill their container. Some elements and compounds can exist as solids, liquids and gases (e.g. ice/water/steam), according to their temperature.
Molecules in solids
Molecules in liquids
Molecules in gases
The atom
Bohr's model of the atom, as we have seen, is similar to our solar system, with the nucleus in the middle and electrons travelling in different orbits called shells. Unlike the solar system, however, which is governed by gravitational forces, the behaviour of an atom is governed by electric forces: i.e. by the attraction and repulsion between electric charges.
Electric charges have been arbitrarily assigned as being either positive or negative, and behave according to a universal law which states that:
• like charges repel each other
• unlike charges attract each other.
Atoms are made up of three types of particles: protons, neutrons and electrons. Protons are positively charged, electrons are negatively charged, whereas neutrons carry no charge at all. The individual protons and neutrons are much more massive than the little electron, but still protons an electrons have equal amounts of opposite charge. Accordingly, electrons are held in their orbits by their attraction towards the protons within the nucleus, which is then exactly balanced by a centrifugal reaction which acts in the opposite direction. Protons are fixed within their nucleus, and all elements are defined by their number of protons. Elements are listed in the Periodic Table of Elements; the simplest element, hydrogen has just one proton in its nucleus; the naturally occurring element with the greatest number of protons is uranium which has 92 protons in its nucleus.
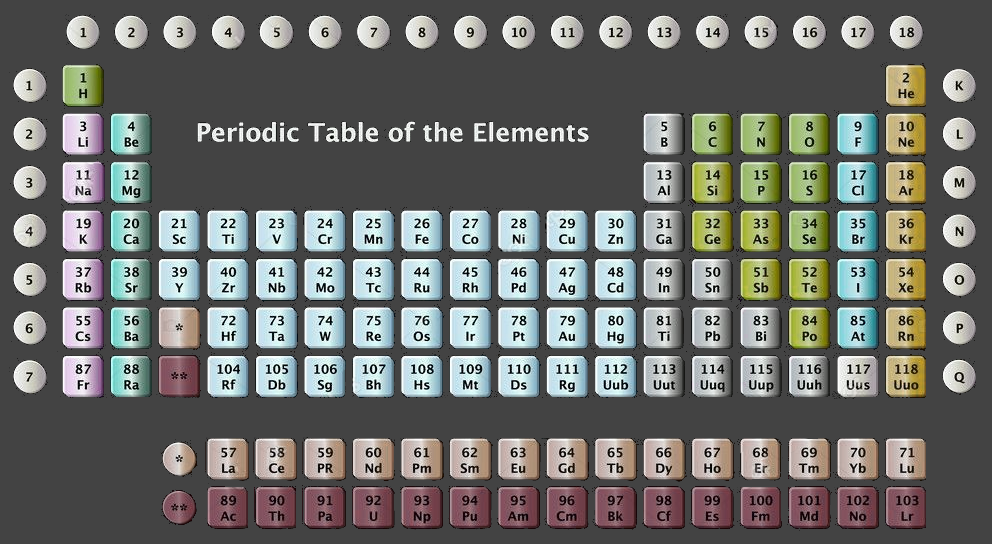
The energy level of each electron determines which shell it occupies. Electrons with the lowest energy level occupy the shell nearest to the nucleus, while electrons with the greater energy level occupy the shell further away from the nucleus.
Ernest Rutherford was absolutely right when he believed that most of the volume occupied by an atom was simply ‘empty space’. To put this into perspective, the diameter of Bohr’s atom is at least 100 000 times the diameter of its nucleus!
The amount of negative charge on one electron is identical to the amount of positive charge on one proton. Under normal circumstances, atoms are neutral and, so, for every positively charged proton, there must be a corresponding negatively charged electron. When we inject enough energy into an atom, we can get electron to pop off being free. The rest of the atom, so, remains positively charged, but as soon as this happens, another electron comes along which takes place in the open slot in atom's shell. The amount of energy or work it takes to pop one of these electrons loose depends od the type of atom we're dealing with. In an insulator these electron charges are "stuck" in shells, orbiting the nucleus of the atom, whereas in a good conductor, electrons in the outer shells of the atoms will pop off at the slightest touch; in metal elements, they bounce around from atom to atom very easily.
In Bohr’s model of the atom, the most complex atoms have as many as seven shells.
The outermost shell in any atom is called its valence shell, and it is this shell that determines an atom’s electrical (and chemical) properties.
Regardless of its electron capacity, the valence shell can actually only support up to eight electrons. So, for example, if the valence shell of a particular atom has a ‘capacity’ of, say, 18, once it is actually occupied by eight electrons, no further electrons can be added. Instead, a new valence shell is formed and, once this new valence shell has acquired eight electrons, the previous valence shell will then be able to continue to build up its capacity to 18.
We said that the valence shell has a maximum capacity of eight electrons. If the valence shell has less than four electrons, then that shell is considered to be unstable – its electrons are loosely secured within that shell and can easily break away from the atom to become ‘free electrons’. Elements with large numbers of free electrons are mainly metallic elements, and are called conductors. Conductors provide the free electrons necessary to support electric current.
If the valence shell contains more than four electrons, then that shell is said to be stable and its electrons are held tightly within that shell. This type of element behaves as an insulator. Although many elements behave as insulators, most practical insulators are actually manufactured from compounds such as plastics, rubber, glass, ceramics, etc.
Ionisation and ions
When an atom’s valence shell temporarily loses or gains an electron, it acquires an electric charge due to the imbalance between its number of electrons and the number of protons contained within its nucleus. We call a charged atom an ion, and the process of losing or gaining an electron is called ionisation.
If an otherwise neutral atom loses an electron (so there are now more protons than electrons), then it acquires an overall positive charge and, so, is called a positive ion and tends to attract a nearby free electron – thus becoming neutral again. So within conductors, both positive ions and free electrons have a very short lifespan! Immediately an electron breaks away from an atom to become a free electron, that atom becomes a positive ion and attracts a nearby free electron, thus becoming neutral once more.
If an otherwise neutral atom gains an electron (so there are more electrons than protons), then it acquires an overall negative charge, and is called a negative ion and tends to repel nearby free electrons.
Electric current
Practical conductors are mainly metallic elements with an abundance of free electrons which move extremely rapidly from one atom to another in various directions.
Suppose we now apply an external positive charge to one end of this length of conductor, and an external negative charge at the other end. Free electrons, being negatively charged, will be repelled by the external negative charge, and attracted towards the positive external charge. There will now be a general tendency for the free electrons to drift from the negative end of the conductor towards the positive end. The quantity of free electrons that drift through a metallic conductor, per unit time, is termed an electric current, and is expressed in amperes (symbol: A). What actually happens is that the electrons continue with their frantic and chaotic movement, but there is a very gradual tendency for them to drift towards the positive end of the conductor.
So, in both cases then, electrons continue to move chaotically and at great speed within the conductor. But, under the influence of external charges, there is a very gradual drift along the conductor. While the effect of this drift of free electrons, due to their mutual repulsion, is practically instantaneous along the entire length of the conductor, the progress of individual free electrons along the conductor is very, very slow. So slow, in fact, that an individual free electron, for example, is unlikely to complete its journey through the length of a flashlight lamp filament during the lifetime of that flashlight’s battery.