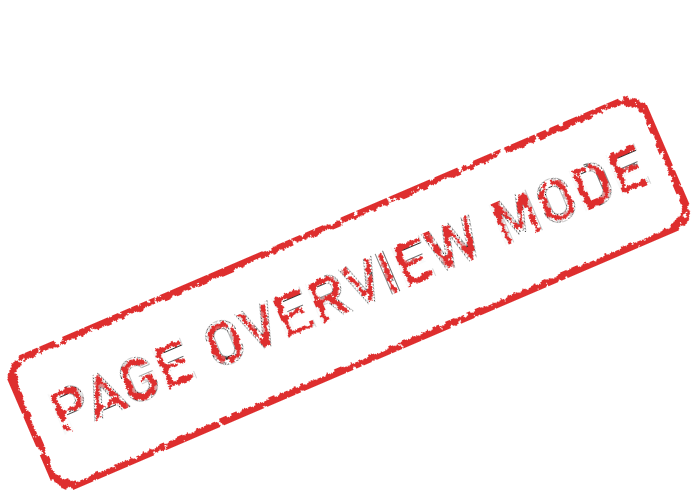
Solar Power Plant
A solar Park is an area of land where many solar panels are installed. A single solar panel can produce a small amount of electricity, but when solar panels are connected in series they form what is called a solar array and it produces usable voltage. In this configuration the positive terminal of one panel is connected to the negative terminal of the next panel creating a chain that increases the voltage output of the array. Now let's take a look at the structure of a single solar array. In larger solar arrays multiple strings of solar panels are connected in parallel. The wiring from each string is then combined in a combiner box which collects the DC electricity from all the strings and directs it toward the next stage of the system. The collected DC electricity is then fed into an inverter which converts it into alternating current AC electricity. This conversion is necessary because most electrical appliances and the grid operate on AC power. The grid serves as an interconnected network that distributes electricity from power plants to consumers ensuring reliable and efficient delivery of power. It facilitates the balancing of supply and demand allowing for real-time adjustments to meet fluctuating energy needs. In this stage high voltage or step-up transformers are used to increase the voltage for transmitting power over long distances. With the help of these towers some solar plants utilize battery banks - used to store excess electricity generated during periods of sunlight for later use when sunlight is unavailable.
Batteries are safely installed inside these cabinets ensuring secure storage and operation. Battery banks are typically installed before or after the inverter stage in a solar power system. Once the electricity generated by solar panels is converted from direct current DC to alternating current AC by the inverter any excess energy can be stored in the battery bank for later use. A solar panel consists of multiple layers designed to protect the panel with solar cells being the primary components. Solar cells are composed of semiconductor material such as silicon. When sunlight penetrates the solar panel it generates electricity typically in the form of direct current DC. Each cell contains two pieces of silicon; one that is negatively charged and another that is positive. When light particles collide with the negatively charged silicon electrons fall and get attracted to the positively charged silicon. As electrons travel from silicon to silicon they create a current which we call direct current electricity or DC. This is the type of electricity found in batteries. A number of solar cells connected to each other and mounted in a frame is called a module. Each module is designed to generate about 30 volts DC. To generate large amounts of electricity modules are grouped together to form an array. These are scattered throughout an open field to capture as much solar power as possible but this electricity is still not ready for public use. It needs to be converted to match the type of electricity needed by home appliances. That's why solar power plants have inverters and transformers. The inverter converts the single current direction of DC electricity to multiple directions of alternating current or AC. The electricity then flows to the transformer and boosts it to high voltage to reduce power loss when electricity is transmitted to far distances. From the transformer electricity flows to a substation. This regulates electricity to usable 220 volts using another transformer and distributes it to power homes.
Wind Power Plant
The basic principle of wind energy is simple. A series of sails or blades mounted around a rotor catch the wind and translate its kinetic energy into rotational energy. Traditional windmills use that rotational energy to grind wheat or pump water. But in modern wind turbines, it turns a generator that creates electricity. This conversion from wind to rotational energy to electricity has defined wind turbines since their invention in the late 19th century. And there are three primary factors that determine just how much energy they can produce: the size and orientation of the blades, the blade’s aerodynamic design, and the amount of wind turning the rotor.
First up, blade orientation. Wind turbines can be designed with their rotor on a vertical axis or a horizontal axis. Vertical blades can pick up wind coming from any direction, but with much less efficiency than horizontal axis rotors. Horizontal designs allow blades to capture the wind’s full force by tracking the wind’s direction and turning to face it. This turning process is called yawing, and older windmills achieved it through manual monitoring. Today, wind sensors and computer systems automatically adjust the blades with expert precision to capture as much energy as possible. Outside rotor orientation, the blades themselves need to be shaped to maximize efficiency. While early designs used flat blades, modern blades are curved like airplane wings. Wind travels faster over the curved surface, creating a low-pressure pocket above the blade that forces it upwards. Since the amount of lift depends on the angle at which the wind is moving relative to the blade, modern blades also incorporate a twist, optimizing how much of the blade can cut into the wind. Made of fiberglass and resin layers, these blades are strong enough to operate through rain, lightning, and blistering sunlight for over 20 years. Even with aerodynamic blades and a horizontal rotor, a wind turbine can only capture wind if it's in a windy environment. Wind speeds typically increase the higher into the atmosphere we travel. So today, most turbines are well over 100 meters tall, with equally large rotor diameters. A turbine of this height and size can capture a huge amount of wind, generating enough electricity every year to power about 750 homes. A wind farm of 200 similarly sized turbines could power over 150.000 homes for an entire year. Wind may be a free and unlimited fuel, but no matter how large or efficient a turbine is there's a mathematical limit to how much wind it can convert into electricity. German physicist Albert Betz calculated that since some wind must remain to keep the blades spinning, a turbine can only ever capture 59.3% of the wind’s energy. Additionally, some people feel turbines disrupt natural scenery, and wind energy’s intermittent availability can make it difficult to integrate into electrical grids. But even with these challenges, modern wind turbines have made wind energy the most efficient and inexpensive source of electricity.
Power plants generate electricity through various technologies that use fossil fuels, nuclear fuels, or renewable energy. Power plants that burn fuels generally use steam boilers, combustion turbines, or both. Steam boilers burn fuel to heat water and produce steam. This steam is then channeled through a turbine, where it turns the blades and generates electricity. Combustion turbines burn fuels to create exhaust gases, which spin the turbine to generate electricity. Some combustion turbines use waste heat from fuel combustion to produce steam to turn the blades of another turbine to generate electricity. These types of power plants are referred to as combined cycle power plants and are more efficient than steam boilers or combustion turbines alone. Many power plants do not burn any fuel to generate electricity. Nuclear power plants are like steam boilers, but the steam is produced from nuclear reactions rather than from fuel combustion. Wind turbines and hydropower use wind or flowing water, respectively, to spin turbine blades that are connected to electricity generators. Solar thermal power plants are like steam boilers, but the steam is produced from concentrated solar energy instead of fuel combustion. Solar photovoltaic (PV) panels absorb light energy from the sun, charging electrons to generate electricity.
After electricity is generated, the electric power grid carries it long distances to residential and commercial buildings and industrial facilities. Electric utilities and grid operators need to ensure that enough power will be generated to meet demand. As the name implies, "baseload” power plants, such as large nuclear and fossil fuel power plants, operate without much interruption throughout the year. Intermediate, or load-following power plants, adjust their electricity output as demand for electricity fluctuates throughout the day. During times of peak electricity demand, "peaker plants" (power plants that only operate during peak demand) can supplement electricity to meet demand. When electricity supply is greater than demand, excess electricity can be stored for later use. As more renewable energy power plants are connected to the electric power grid, energy storage technologies (e.g., batteries, pumped storage) play a more important role in the electricity system as it helps align renewable energy generation produced in off-peak hours with period of higher electricity demand. The system must balance base load and peak load demands.
Base Load: This is the minimum level of demand on an electrical grid over a 24-hour period. Base load power plants, like nuclear, coal, and hydroelectric plants, run continuously to meet this constant demand.
Peak Load: This is the maximum demand during a specific period, often short-term and higher than the base load. Peak load plants, like gas turbines and some renewable sources, are activated during these high-demand periods.
Energy storage is crucial for balancing supply and demand. We can have differrent methods, like:
1. Pumped hydroelectric: Electricity is used to pump water up to a reservoir. When water is released from the reservoir, it flows down through a turbine to generate electricity.
2. Batteries: Similar to common rechargeable batteries, very large batteries can store electricity until it is needed. These systems can use lithium ion, lead acid, lithium iron or other battery technologies.
3. Thermal Energy Storage: Electricity can be used to produce thermal energy, which can be stored until it is needed. For example, electricity can be used to produce chilled water or ice during times of low demand and later used for cooling during periods of peak electricity consumption.
4. Flywheels and Compressed Air: Electricity is used to accelerate a flywheel (a type of rotor) through which the energy is conserved as kinetic rotational energy. When the energy is needed, the spinning force of the flywheel is used to turn a generator. Some flywheels use magnetic bearings, operate in a vacuum to reduce drag, and can attain rotational speeds up to 60,000 revolutions per minute. Electricity is used to compress air at up to 1,000 pounds per square inch and store it, often in underground caverns. When electricity demand is high, the pressurized air is released to generate electricity through an expansion turbine generator.
Frequency in power systems must be kept stable (usually around 50 or 60 Hz) to ensure reliable operation. When demand exceeds supply, frequency drops, and vice versa. Power plants adjust their output to match demand and maintain frequency. Storage systems can quickly respond to frequency changes by either absorbing excess energy or supplying energy as needed.